CMPL SEMICON
National Institute of Physics
University of the Philippines - Diliman
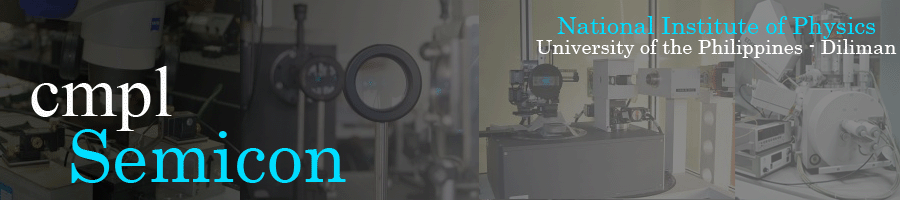
The quality of a solar cell is usually measured in terms of its ability to absorb and convert light into electricity, termed as conversion efficiency. One of the key challenges in fabricating solar cells is to develop techniques and materials that can maximize conversion efficiency of the cell. Utilizing semiconductor materials that can absorb most of the visible light is one of the potential solutions in increasing the conversion efficiency of a solar cell. The Condensed Matter Physics Laboratory is currently conducting research on new materials that can serve as light absorbing layers in solar cells. These include zinc oxide, titanium oxide, copper oxide, and copper nitride.
Solar Absorbers
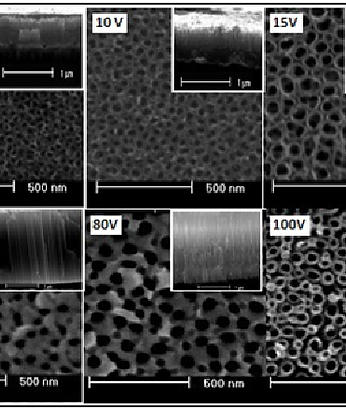

Cuprous oxide (Cu2O) is a metal oxide that is recently becoming a popular material in semiconductor physics. Its wide energy gap of 2.1 eV has made it suitable for photovoltaic applications. Recent studies have shown its capabilities as a possible new material for commercial solar cells. Cu2O has been projected to serve as a low cost alternative to more commonly used materials like silicon and gallium arsenide. It is also one of the very few semiconductors that exhibit p-type conductivity. The theoretical limit for the energy conversion efficiency of a Cu2O-based solar cell is around 20%. As of 2013, the highest reported efficiency is only around 3%, leaving opportunities to maximize its potential as a solar cell material open.
The fabrication high-purity Cu2O through thermal oxidation of copper (Cu) foils are currently being studied at CMPL. Studies are focused on determining the light absorption properties of Cu2O and also on its applications. Cu2O films grown via thermal oxidation are shown to have a generally rough appearance characterized by the presence of grains with varying sizes. X-ray diffractometry was done on the Cu2O films to inspect their quality. on semiconductor devices. Scanning electron microscope (SEM) images of Cu2O film grown on Si are shown in the Figure.
Reflectance spectra of Cu2O grown on textured Si and its parent textured Si substrate are shown in the figure below. The reflectance spectra of the Cu2O-coated textured Si roughly mimic the spectrum of the textured Si, albeit with decreased reflectance values in the region of visible light. This indicates that Cu2O enhances the light absorbing capabilities of the sample while preserving the spectrum of the Si substrate. A device based from a Cu2O-ZnO heterojunction has been fabricated to demonstrate the capabilities and potential of Cu2O as a new solar cell material. The diode was fabricated through the following steps: (1) deposition of a layer of fluorine-doped tin oxide (FTO) on a cover slip


Titanium dioxide is a semiconductor material utilized in a wide range of applications due to its unique and useful properties like its wide band gap and photocatalytic properties. Titanium dioxide is often used as a component in solar cell devices, primarily in dye-sensitized solar cells (DSSCs). Conventional titanium dioxide thin films, however, does not yield high photoconversion efficiencies because of their limited surface area therefore nanostructured titanium dioxide thin films composed of layers of nanoparticles or nanotubes are often used.
Titanium dioxide nanotubes can be fabricated using different techniques such as electrochemical oxidation or anodization, sol-gel method, hydrothermal method and template-based methods. Among these techniques, anodization is the most economical and simplest method of producing uniform self-organized and tunable titanium dioxide nanotube arrays. The growth of titanium dioxide nanotubes, via anodization, can be done using a simple galvanic setup composed of a metal cathode, the anode which is the growth sample, an electrolyte and a stable power supply. The properties of titanium dioxide nanotubes, both optical and electrical, are affected by their morphological features. It is essential that these features can be controlled during the growth process in order to produce nanotube arrays with properties suitable for specific applications. By varying different anodization parameters, the morphology of the nanotubes can be controlled.
The electrolyte used during anodization as well as the duration of anodization primarily serves as limiting factors for the length of the grown nanotubes. Nanotubes grown in HF-based electrolytes tend to have limited tube lengths which can barely reach about 1 micron. On the other hand, the tube length of nanotubes grown in ammonium fluoride based electrolytes can easily reach a micrometer and achieve a length exceeding a millimeter8. The anodization voltage sets the rate of nanotube growth, thus greatly affect
the diameter as well as the length of the grown nanotubes. The length and diameter of the nanotubes increases with the increase in voltage used during anodization. This trend is observed in samples grown in HF and ammonium fluoride-based electrolyte.
Copper Oxide

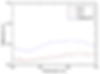
Titanium Dioxide Nanotubes
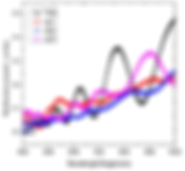
